In the previous tutorial, we learned that the modular design method is effective for developing a highly reliable, high-performance power distribution network (PDN) that can handle different specifications in a limited amount of time. showed. Also, in the second half, I explained how to design a filter, which is necessary when developing a DC-DC system using a switching power supply module.
The third tutorial will cover stability issues caused by filter components and distribution line impedance due to the interconnection of power sources and power modules.
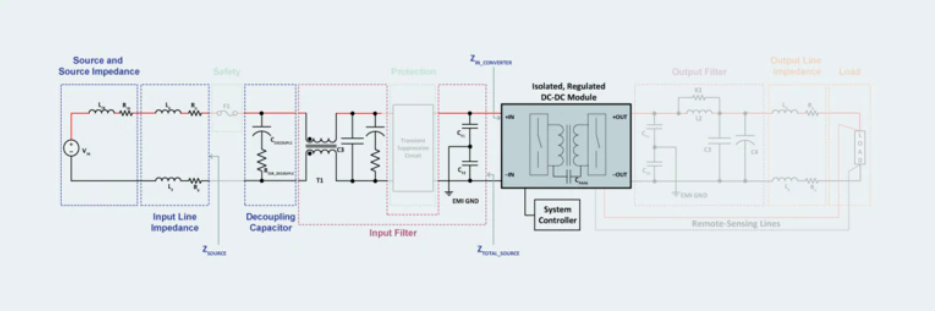
Figure 1: Designing a complete power system around a DC-DC converter module requires a filter circuit.
Adding a filter introduces system stability problems, so decoupling the power source and the converter should be taken care of.
Principles of stability analysis
To analyze the stability of the entire DC-DC converter system, first divide it into two subsystems: the power source and the load. Here, the "load" subsystem is the DC-DC converter itself. The power source impedance, input line impedance, and input filter are part of the power source subsystem.
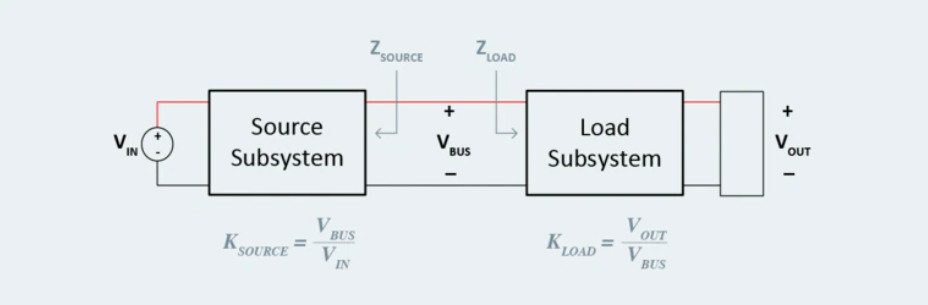
Figure 2: Block diagram representation of ideal interacting power source and load subsystems for stability analysis.
The source and load subsystems are each considered inherently stable. However, when the two are connected at VBUS, various interactions can cause oscillations and system instability.
When analyzing the stability of this type of system, be aware that the impedance of the power source seen by the DC-DC converter becomes a finite, frequency-dependent value due to the input filter and the components installed in the previous stage. is needed. A source subsystem with finite output impedance feeds a load subsystem that also has finite input impedance, and the impedance ratio is a key factor in analyzing system stability. element. The system is stable only if the input impedance of the load subsystem is greater than the output impedance of the power source subsystem, as also found in the Middlebrook stability criterion.
The ratio of this impedance is defined as the minor loop gain of the system. That is, the power source impedance looking into the power source subsystem (including the input filter and all connected impedances and the output impedance of the power source itself) versus the input impedance looking into the DC-DC converter.
Based on this relationship, the ratio (TMLGMore).To ensure system stability, the power source impedance should be less than the load impedance.
Exceeding this condition can lead to poorly damped circuits and highly unstable systems. In practice, a negative resistance is formed between the power source and the DC-DC converter, whichcan cause the system to oscillate, which is extremely problematic for system operation.
Decoupling the input section of the converter and the power source impedance
Decouple the power source and load impedances so that oscillations do not destabilize the system. This can be effectively achieved by placing a decoupling capacitor on the input side of the DC-DC module. For clarity, Figure 1 shows a simplified power source represented by a Thevenin equivalent circuit (ideal power source and discrete impedance blocks). Additionally, the impedance of the wiring leading to the DC-DC converter (consisting of inductance and resistance) is also part of the power source subsystem.
Separate the system with a bus between the DC-DC converter and the power source to clarify the output impedance looking at the power source side and the input impedance looking at the DC-DC converter side. For simplicity, the input impedance of the DC-DC converter is assumed to be resistive only and fixed at all frequencies. It is at this low to high frequency transition that the stability analysis is important. This is because the output impedance of the power source subsystem, which has the characteristics of inductance, increases.
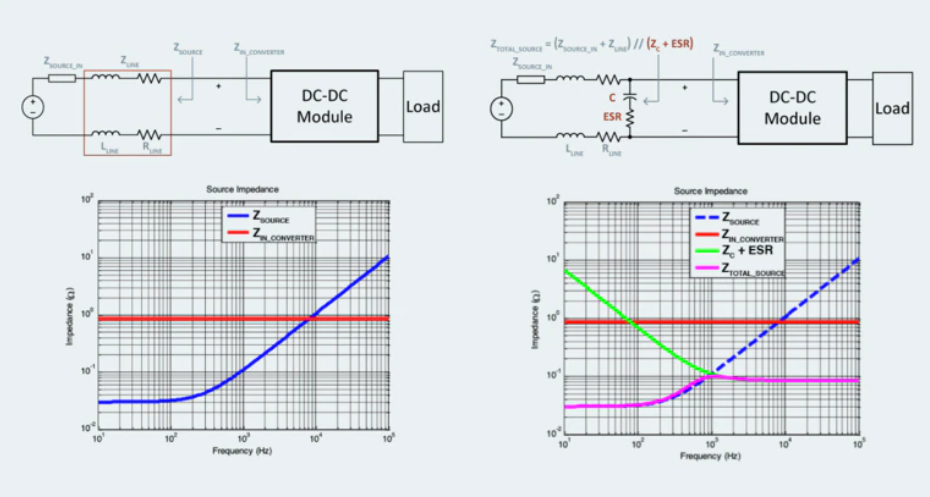
Figure 3: By inserting a capacitance with ESR, the power source impedance becomes lower than the input impedance of the DC-DC converter even in the high frequency range, ensuring the stability of the entire system.
In order to ensure stability in the high frequency range, it is necessary to adjust the power source impedance that connects the DC-DC converter. A relatively easy method is the element of damping. Adding capacitance and equivalent series resistance (ESR).
A capacitive impedance (green line) inserted between the output impedance of the power source and the input impedance of the DC-DC converter decreases with increasing frequency, bypassing the inductance component of the power source. Therefore, the equivalent output impedance on the power source side can be lowered over the low to high frequency range in which the DC-DC converter operates.
Input Filter and System Stability
Adding an input filter to a DC system can change the output impedance of the power source and cause system instability. Adding filter elements can lead to system instability or oscillation if the Middlebrook stability criterion is not met. In an unstable system, load fluctuations can cause oscillations, creating unwanted input bus voltage transients.
When defining the input impedance of a DC-DC converter with DC-DC voltage regulation, the operating conditions of the system are minimum input voltage and maximum input power. Then, as in the previous example, we get an approximation of the resistive component of the input impedance of the DC-DC converter. System stability can be evaluated by comparing the resistance approximation of the input impedance of the DC-DC converter with the output impedance of the power source (including additional filter elements).
The analytical example in Figure 4 shows an initial input filter design with a resonant peak of the power source impedance between 10kHz and 11kHz. Due to this resonance peak, we can see that there is some interaction between the power source impedance and the load impedance. This is due to the lack of damping on the frequency response of the power source combined with the input filter. In this frequency region, the impedance of the power source is greater than the impedance of the load, so system instability can be expected.
However, with proper damping, the resonance peak can be reduced, and the input impedance of the DC-DC converter and the output impedance of the power source can be properly decoupled. can satisfy With proper damping of the filter circuit, the system can avoid unwanted oscillations and instabilities, resulting in good overall performance.
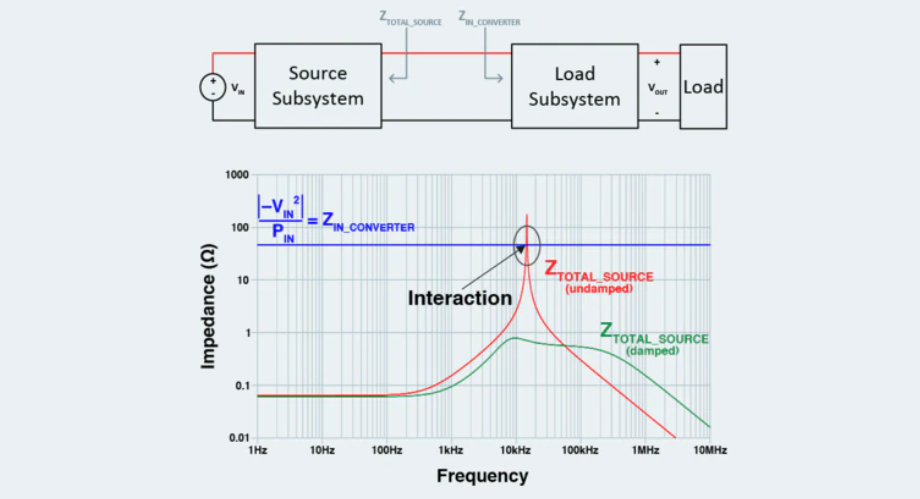
Figure 4: When adding an input filter to the system, it is necessary to perform impedance analysis assuming the worst value of the input impedance of the DC-DC converter and adjust the damping to ensure stability.
Once the basic system design is complete, move on to the next stage
At this stage, the design of the DC-DC system has met the requirements of the application. The architecture and the DC-DC module were decided, and appropriate filters were added to reduce the effects of module noise, ensuring the stability of the entire system assuming worst-case conditions in the high-frequency range. Now that a stable and effective system has been established, the next design phase is to protect the system from failure-causing transients and from catastrophic failures, including the surrounding system.
We will explore this in the next tutorial.